25 August 2016
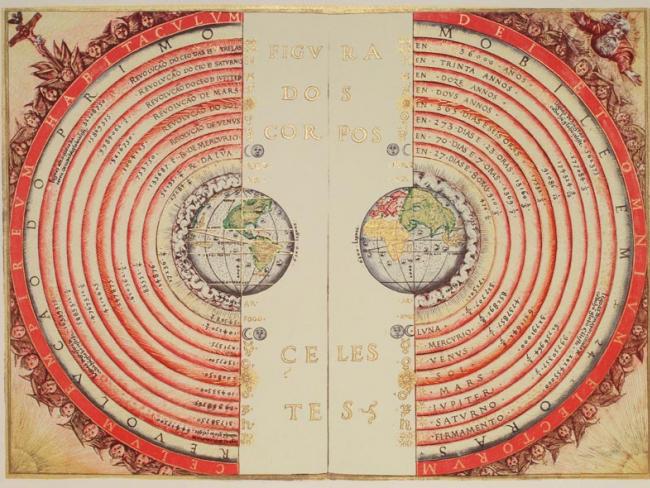
How the Earth used to be viewed: an illustration by Portuguese cartographer Bartolomeu Velho, now in the National Library in Paris.
400 years ago the Catholic Church banned Copernicus’s books and announced its first judgement against Galileo…
In the late sixteenth and early seventeenth centuries two men helped to cast out ancient ideas about physics and astronomy. Their work laid the foundation for modern scientific understanding.
Copernicus (1473-1543) was a Renaissance mathematician and astronomer, who studied astronomy and maths at Krakow University and obtained a doctorate in canon law (church laws and regulations). He studied medicine at Padua University, and then practised it for nearly half a century. He spent his last decades at Warmia (now in northern Poland) observing and developing his theories on astronomy.
His central theory was that the earth rotates daily on its axis and revolves yearly around the sun, as do the other planets. This was a heliocentric, “sun-centred” system (from the Greek word helios meaning “sun”). It challenged the long held opinion that the Earth was stationary at the centre of the universe with all the planets, the Moon and the Sun rotating around it.
Copernicus probably discovered his main idea sometime between 1508 and 1514, when he wrote a manuscript entitled the Commentariolus (meaning “Little Commentary”). Even though the final version of his theory On the revolutions of the Celestial Spheres was essentially finished by 1532, the book did not appear in print until 1543, the year of his death.
New ideas
A heliocentric system went against the accepted view of his day. Copernicus tentatively shared his ideas in Commentariolus with selected friends. He continued to think, recalculate and gradually develop the treatise that would fundamentally revise the understanding of cosmology and physics held since classical Greek times.
Copernicus delayed publication, possibly fearing academic and religious opposition, and waited thirty years to issue the mature, evidentially-rounded version of his heliocentric theory. It was published in 1543 in the receptive town of Nuremberg, a centre of business, finance, enterprise and technology. Interestingly, no one was punished or threatened; his book was read in universities across Europe. A second edition appeared in 1566. His treatise was not proscribed, probably because it included lots of maths and he wrote it in Latin. There was no posthumous proclamation of Copernicus as a heretic; no hunting down or punishing of those who agreed with him.
‘Copernicus’s theory was a challenge to two thousand years of physical science.’
The church was slow to condemn Copernicus’s ideas. Heliocentricism was even being lectured upon in Rome by the Papal Secretary when Pope Clement VII (1523-34) was part of the audience. Copernicus’s heliocentric theory only became a matter of theological contention with the warning given to Galileo in 1616, 73 years after its original publication.
To the traditionalists the heliocentric system seemed to be absurd and contrary to common sense, as the earth did not appear to be flying through space and spinning on its axis as well. Copernicus’s theory was a challenge to two thousand years of physical science represented by the philosophy of Aristotle and medieval logical analyses of motion.
Observations
The test of Copernicus’s theory lay not in brilliant rhetoric or philosophical argument, but in empirical observations. He made copious measurements of the exact angular position of astronomical bodies, undertaken with instruments of increasing accuracy.
Copernicus’s development of a heliocentric theory of the cosmos arose from a desire to make sense of the universe and to devise coherent explanations for the movement of the planets. Philosophers in the classical tradition did not for the most part understand the need to match theories and observation.
Born 21 years after Copernicus’s death Galileo Galilei (1564-1642) was an astronomer, mathematician, philosopher, physicist and engineer. Considered the father of modern science by many, including Albert Einstein and Stephen Hawking, he is renowned for his studies on the laws of motion and for many scientific discoveries. These include the telescopic confirmation of the phases of Venus (which validated Copernicus’s theory), the discovery of the four largest satellites of Jupiter and the observation and analysis of sunspots.
Starting a medical degree at Pisa University, he quickly found it was not to his taste and studied mathematics and natural philosophy instead. In 1589 he became professor of mathematics. From 1592 to 1610 he taught geometry, mechanics and astronomy at Padua University, which at the time was not only Italy’s but probably Europe’s top university for scientific and medical subjects.
Galileo pioneered not only the development of the telescope as an astronomical instrument, but also an early microscope – and was amazed at the complex beauty of insects. His Starry Messenger (1610) was the first published scientific treatise to be based on observations made with a telescope. He exploited the international acclaim which his 1610 telescopic discoveries brought him to secure a prestigious appointment at the court of the Grand Duke of Tuscany.
Condemnation
In 1614, Galileo was accused by the Roman Catholic Church of heresy for his support of the Copernican theory that the Sun was at the centre of the solar system. In 1616, the church banned him from teaching or advocating these theories. He was again condemned for heresy in 1632 for defending his views and the Copernican theory in his book Dialogue Concerning the Two Chief World Systems.
Galileo was summoned to appear before the Inquisition in Rome. He was convicted, forced to recant and publicly withdraw his support for Copernican theory. He was sentenced to life imprisonment, later reduced to permanent house arrest which remained the situation until his death.
For the church, Galileo’s main fault was not to argue for his idea (which was not unusual and often tolerated within learned confines) but to publish his work in everyday Italian, so that non-scholars could read it. As the ruling classes have always realised, ideas become a material force once they are grasped by the mass of people, and then become a danger to ruling class power.
Though going blind, Galileo continued to write and, in 1638, published Discourses Concerning Two New Sciences explaining his ideas on the laws of motion and the principles of mechanics.
In his play Life of Galileo, the German playwright Bertold Brecht (over 300 years late and safe) condemns Galileo for recanting because it removed Galileo’s ideas from public discourse. Yet Galileo had already defied the church once; there is no record of him privately changing his mind and the ideas were already out in the world. There is the tale, which cannot be confirmed or denied historically, that after his recantation he uttered something along the lines of “And yet it [the Earth] moves”. It certainly is very much in keeping with the man: Galileo was a very argumentative, disputatious person.
‘Galileo laid the foundations for a revolution in scientific understanding.’
Galileo had a gift for pure mathematics and applied and mechanical engineering design. Evidently he was a skilled practical operator, good with his hands, at home in the worlds of university academic and practical mathematician, with one foot firmly planted in the world of the tradesman and skilled artisan. He also worked in applied science and technology, inventing instruments.
Galileo was well-read and familiar with the work of his predecessors over the previous 250 years: those late medieval “physicists” who were interested in the relationship between impetus, motion, acceleration, and deceleration.
His work replaced old, untested ideas about motion with objective, verifiable mathematical descriptions. This laid the foundations for the revolution in scientific understanding led by Isaac Newton (born the year Galileo died).
Experiment
Galileo’s approach to all aspects of physics and astronomy was to experiment and observe first, theorise second, and then to describe this mathematically. Galileo epitomised a new approach to understanding the natural world, by the use of controlled experimental conditions, investigating a small part of nature in the hope of gaining insight into the whole.
Though both Copernicus and Galileo probably deserve the title of genius, they were not in any way special figures who saw further than everybody else or grasped the truth while the rest floundered in ignorance. Rather Copernicus and Galileo flourished in an environment increasingly conducive to breakthroughs in knowledge. They benefited from a European tradition of improving technology that went back to the eleventh century.
‘Copernicus and Galileo flourished in an environment conducive to breakthroughs in knowledge.’
Many astronomers of the long Renaissance (1500–1700) devised a series of instruments that transformed the science of classical antiquity amid enormous changes in the wider society that bred perfect conditions for pioneering research.
The ocean-going sailing ships of the Renaissance overturned classical geography by discovering continents and oceans that the ancient Greeks had never dreamed of. These new lands and waters were surveyed and mapped; the results were published by the new-fangled printing presses. These events initiated a rapid development of practical geometry, precision instrument making and cartography.
From 1450 the advent of printing led to a cascade of information and new learning, not only standardising typography for readers and scholars across Europe but also facilitating the mass production of ideas, mathematical calculations, tables, woodcut illustrations and diagrams, and descriptions of instruments within the world of learning. Copernicus read, marked and assimilated much of this material.
Inventions
Practical optics and the study, manipulation and use of light also advanced rapidly. From the late sixteenth century onwards the glass-maker and lens-figurer began to improve upon the original thirteenth-century technology of their crafts. This optical technology would produce two of the most far-reaching human inventions, the telescope and the microscope. They revealed not only the telescopic realm of the universe but also the microscopic realm of exceedingly tiny things.
Precision instruments enabled researchers to detect, measure and quantify things in nature that would be oblivious to our unaided senses. These “artificial organs” were soon directed to the craters on the moon, the individual stars that made up the Milky Way, microscopic “animalcules” in body fluids, barometric pressure and meteorological changes.
Many natural forces, once thought to be mysterious, were measured and quantified. By 1665, there were instruments for the physical study of the earth’s magnetic field (compass and dip-needle), atmosphere and weather (barometer, thermometer, hygrometer), and of combustion, flame, and organic respiration (air or vacuum pump).
The astronomical renaissance was a response to the floodtide of new factual data provided by explorers in ships, from observatories and laboratories, rather than from a deliberate radical philosophy intent on breaking classical and medieval knowledge.
Hands and Minds
Though both Copernicus and Galileo undoubtedly had great minds, crucially they had perceptive eyes, dexterous hands and a distinct practical bent which got the most out of the technology of their scientific instruments. As the scientific ideas of Copernicus and Galileo took hold, many ancient cultural beliefs such as astrology and alchemy bit the dust and essentially the modern world began.
The ancient thinking had lasted for a good two millennia in physics, astronomy and medicine. That was not because it was somehow enforced to stop people thinking for themselves, but because it appeared then to be common sense, the best fit for the then available facts. Even when Copernicus and Galileo were students, their lecturers and teachers were still guided by these views.
Astronomical ideas had developed even before the Renaissance. Some people realised we didn’t live on a flat earth. In the sixth century BC, Pythagoras worked on the assumption that the earth was spherical. Two hundred years later Aristotle observed key things about the earth: that a ship gradually disappeared from view because of the earth’s curvature; that the shadow cast by the earth upon the full moon at a lunar eclipse was always circular. In the third century BC, the Greek mathematician and astronomer Aristarchus of Samos was the first to propose a heliocentric system, though his ideas were not developed beyond a basic outline.
The ancient Greeks invented practical astronomy, devised the earliest known scientific instruments, and, through a combination of observation, measurement, mathematics, and theoretical deduction, came up with an Earth-centred model of the cosmos which would endure until Galileo’s time. Later Ptolemy theorised that the Earth was a large sphere set at eternal rest in the centre of nine perfectly transparent “crystalline” spheres that nested concentrically inside each other rather like the skins of an onion, and which rotated around a common polar axis. A Terry Pratchett world!
However, all these people lacked instruments of sufficient accuracy to measure the very tiny angles involved, but through their grasp of geometry, their attempted observation and measurement, and logical deduction, the ancient Greeks set astronomy on the right lines.
Moon
In these intervening centuries, the Moon had been the biggest difficulty of all, for it moved in an extremely complicated orbit that seemed to come full circle in a period of just over eighteen years. The behaviour of the planets was baffling too. Mars, Jupiter and Saturn appear at certain times to move backwards from the normal direction (retrograde motion). Mars made two such loops for every one of its rotations around the earth, yet Jupiter made twelve, and Saturn nearly thirty.
Copernicus’s heliocentric system supplied an elegant geometrical model for explaining these loops, based upon the idea that the earth moved faster than Mars, Jupiter, and Saturn, and slower than Mercury and Venus, while the Moon simply revolved around us as a satellite.
Science was not entirely absent from medieval Europe. It was actively practised in a few places, particularly a few famed monasteries. As each of the religions required key festivals such as Passover, Easter, and Ramadan to be celebrated in given parts of the year, astronomical observation was important. Astronomers and mathematical calculators, who knew how to get the right answers, were needed. But the lunar and solar cycles are not synchronised; there are twelve complete lunar cycles and eleven odd days in a solar year.
Errors
The length of the solar year adds to the mathematical tangle; an awkward 365 days 5 hours 48 minutes and 46 seconds. This was of no real consequence in the short run. But glaring errors began to emerge as the centuries rolled on: calendar dates did not align with astronomical events such as the solstices and equinoxes. Several Calendar Commissions were inaugurated by successive Popes. For instance, in 1513 Pope Leo X invited the young Copernicus to give expert advice.
Classical astronomy and science also entered the Arab world in the wake of Islam’s expansion after AD 622 across and beyond Arabia. Many Muslim scholars translated astronomical, medical, optical and other texts into Arabic from their original languages. Between AD 850 and 1250 they were fascinated by mathematical and computational astronomy, making significant advances in celestial mapping and the observations of planetary motion.
This development had stalled by the fourteenth century. In 1449 the last great Islamic scientific centre, the Samarkand Observatory, ceased to exist. However by then the academic study of astronomy was already well established across Europe.
Muslims had translated classical Greek texts into Arabic. The expulsion of Islam from southern Italy and Spain led European scholars in the new universities of Paris, Oxford, Bologna, Cambridge and elsewhere to translate these texts into the Latin of medieval Christian scholarship. They descended upon libraries in Sicily and Spain that held Arabic-translated and Greek classical texts.
These scholars learned Arabic to translate “old books” on astronomy, medicine and optics into medieval Latin. They learned Greek to study the original texts in a pure, uncorrupted form which led to a flood of “new” books that surged into Europe. Medieval undergraduates were taught astronomy, basic celestial mechanics, the geometry of eclipses, and more, all within the “common-sense” science of the earth-centred universe, the crystalline spheres and the physics of Aristotle.
Legacy
This complex legacy of classical antiquity and early Arabic thought combined with the heritage of medieval Christendom helped forge ripe conditions for a new understanding to emerge. Copernicus himself was a recipient of a humanist Renaissance education based on original Greek and Latin languages. It involved study of the arts of language and the techniques of understanding: grammar, rhetoric and logic and included the four arts of mathematics and proportion: astronomy, geometry, arithmetic, and music.
This education was designed to train and discipline the mind, to make it logical, balanced, rational and precise. That’s quite the opposite to the superstition and dark ignorance popularly perceived to characterise the Middle Ages.
Teaching would have been in Latin, the international tongue of learned Europe. Prowess in languages was part of the Renaissance academic curriculum. Copernicus was fluent in Polish, German, Italian, Latin and Greek. He was likely to be familiar with the ideas of Ptolemy, other classical Greeks, Arab astronomers and the thirteenth century polymath Ibn Tusi.
Ultimately, the actions of the church against Galileo and Copernicus proved futile. They did not stop the spread and increasing acceptance of the astronomers’ new evidence-backed theories. Innovative ideas appear when prevailing theories no longer adequately explain the new body of data that is emerging.
A shorter version of this article was published in Workers September/October 2016 edition.